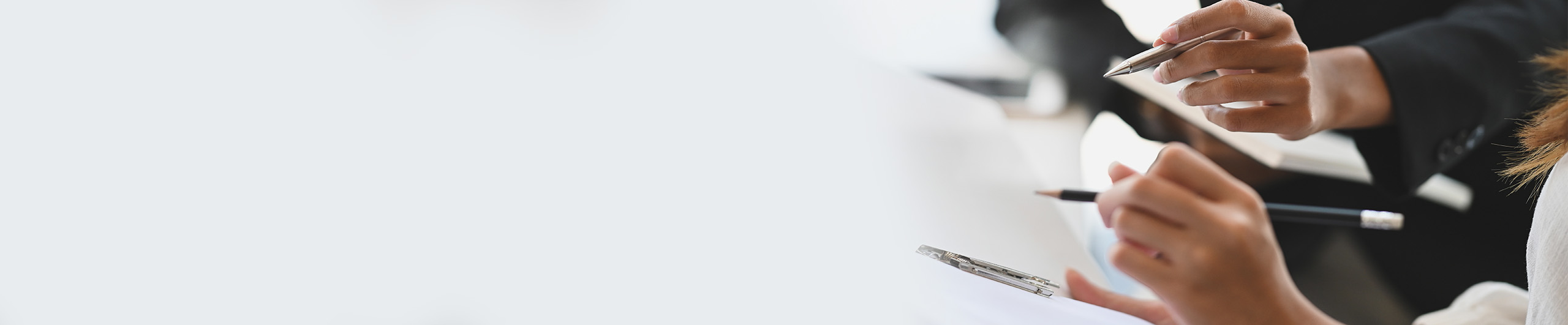
What is Shape Memory Alloy?
1. What is Shape Memory Alloy?
Shape memory alloys are unique materials. After they can be deformed into any shape, it recovers to the original shape before deformation when it is heated above the transformation temperature of the alloy. The stress-strain curves shown in Fig. 1 illustrate this phenomenon. (a) shows mechanical performance of an ordinary metallic material. When it is deformed beyond the elastic range and unloaded, permanent deformation remains. In the case of shape memory alloy (b), the material returns to its original position when heated. (c) shows a “super-elastic” material that returns to its original position after being deformed and unloaded.
Fig. 2 shows the stress-strain curves on the cold-worked and heat-treated alloy at different testing temperatures. At the low temperature range of T≦298K (25℃), a large strain remains, but it disappears and the material returns to the original position when the alloy is heated above the Af point (Austenite phase transformation finished temperature). The shape memory effect appears in this temperature range.

Fig. 1 Stress-Strain curves of Shape memory and
Super-elastic materials
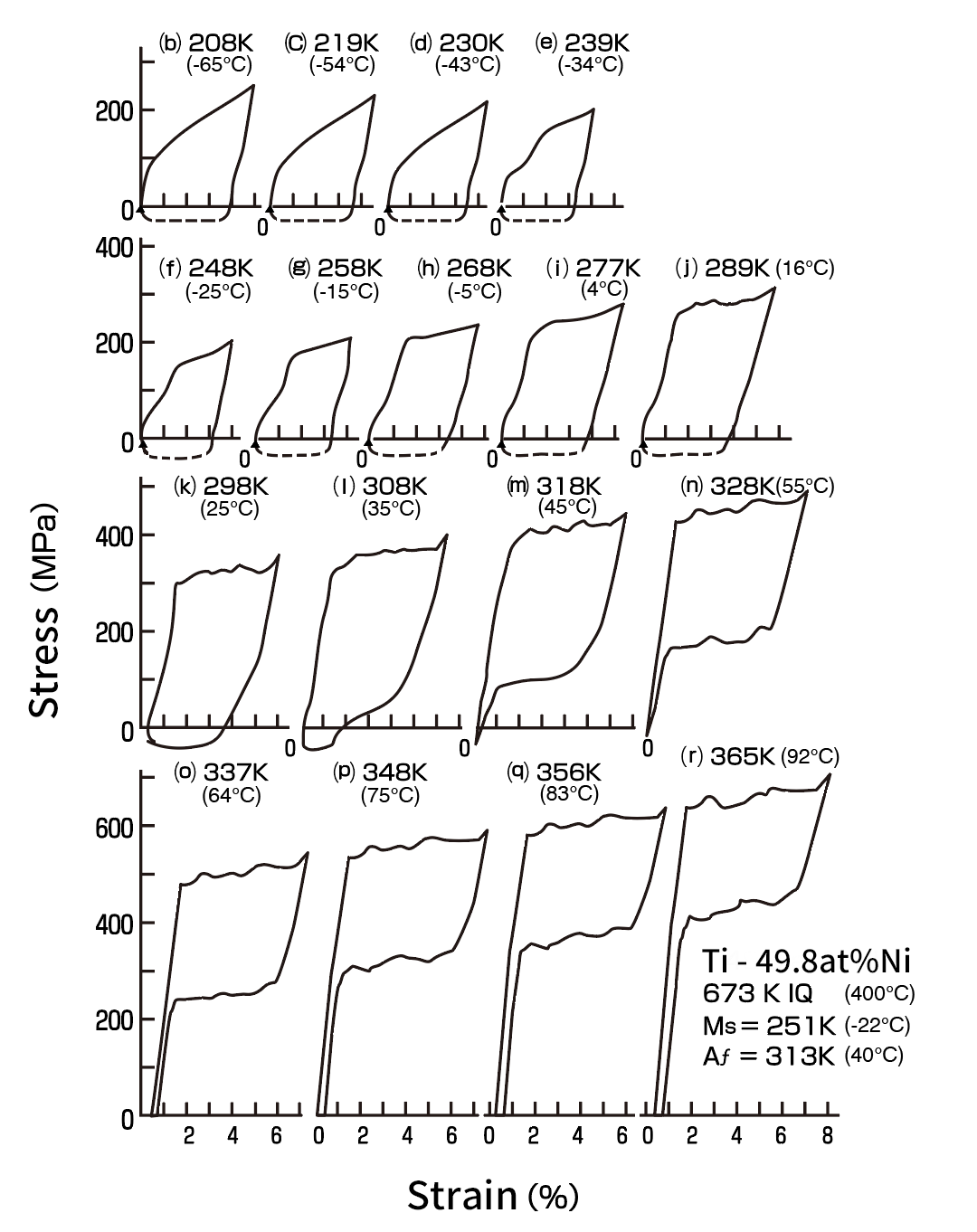
Fig.2 Temperature dependence of stress-strain curve of Ni-Ti alloy
*The dotted line in the figure represents the shape recovery
when the sample is deformed and then heated above the Af point
On the other hand, the stress-strain curve at T≥318K (45°C) shows that the material returns to its original position like rubber when unloaded. This phenomenon observed at high temperatures above the Af point is called ”Super-elasticity”.
Fig. 2 shows that the phenomena (b) and (c) in Fig. 1 appear at different temperature ranges in one material. The shape memory effect and super-elasticity are caused by the phase transformation from the parent phase (Austenite phase) to the Martensite phase and from the Martensite phase to the parent phase. A schematic diagram of this phenomenon at the atomic level is shown in Fig. 3.
In general alloys, loading causes slips in certain planes of crystal structures, as shown in (a)②, and the slips generate shear strain, as shown in (a)③. This slip deformation does not change the crystal structure.
In shape memory alloys, when the parent phase (b)① is cooled below the transformation temperature, the crystal structure changes to Martensitic phase as shown in (b)②. When a load is applied to the material in this state, the neighboring atoms are connected (without breaking) as shown in (b)③, and the material deforms along the loading stress. When the material is heated above the transformation temperature, it undergoes a reverse transformation to the parent phase and it returns to the original shape shown in (b)①. This is the ”Shape Memory Effect”.
When the parent phase (c)① is deformed at a temperature above the Af point, the Martensitic phase is induced by external forces and deforms as shown in (c)②. When the external force is removed, the Martensitic phase, which is energetically unstable, undergoes a reverse transformation to the parent phase and it returns to the original shape of (c)①. This is the ”Super-elasticity”. The recovery strains are up to 7-8% in Ni-Ti alloys. In general, materials with an Af point below room temperature are called as “Super-elastic alloys”, which exhibits super-elasticity at room temperature.

Fig. 3 Schematic diagram showing the movement of atoms
and shape change of crystal structure in various deformation
modes and temperature changes.
Ni-Ti alloys, also known as Nitinol, some copper-based and iron-based alloys are used practically in the market. Ni-Ti alloys are by far the most superior in terms of shape recovery properties and its durability.
The measurement of the transformation temperature is important in evaluating and analyzing the properties of shape memory effect and super-elasticity. The principle of the DSC(Differential Scanning Calorimetry), which is a typical method, is shown in Fig. 4. The start and finish points of the exothermic peak during the cooling process of the sample are determined as the intersection of two tangent lines and defined as Ms and Mf, respectively. Similarly, As and Af are obtained from the endothermic peak in the heating process.
The transformation temperature measurement of Ni-Ti alloy is generally done at the ingot stage after melting and casting to evaluate its properties as a shape memory alloy, but it is also used to evaluate the transformation temperature in the finished product after cold-working and heat treatment. Fig. 5 and 6 show examples of DSC charts of the ingot and product.
Ni-Ti alloy is well known as a typical alloy with shape memory and super-elasticity properties, and many applications have been developed and put into practical use. The transformation temperature can be consciously controlled from 0 to 100°C by slightly changing the Ni-Ti ratio around the atomic ratio of 50:50. It is also available to add some elements to the Ni-Ti binary alloy to give it the characteristics required. Cu, Co, Cr, Fe, etc. are commonly used as the elements.

Fig. 4 Definition of transformation temperatures
on DSC (Differential Scanning Calorimetry) method
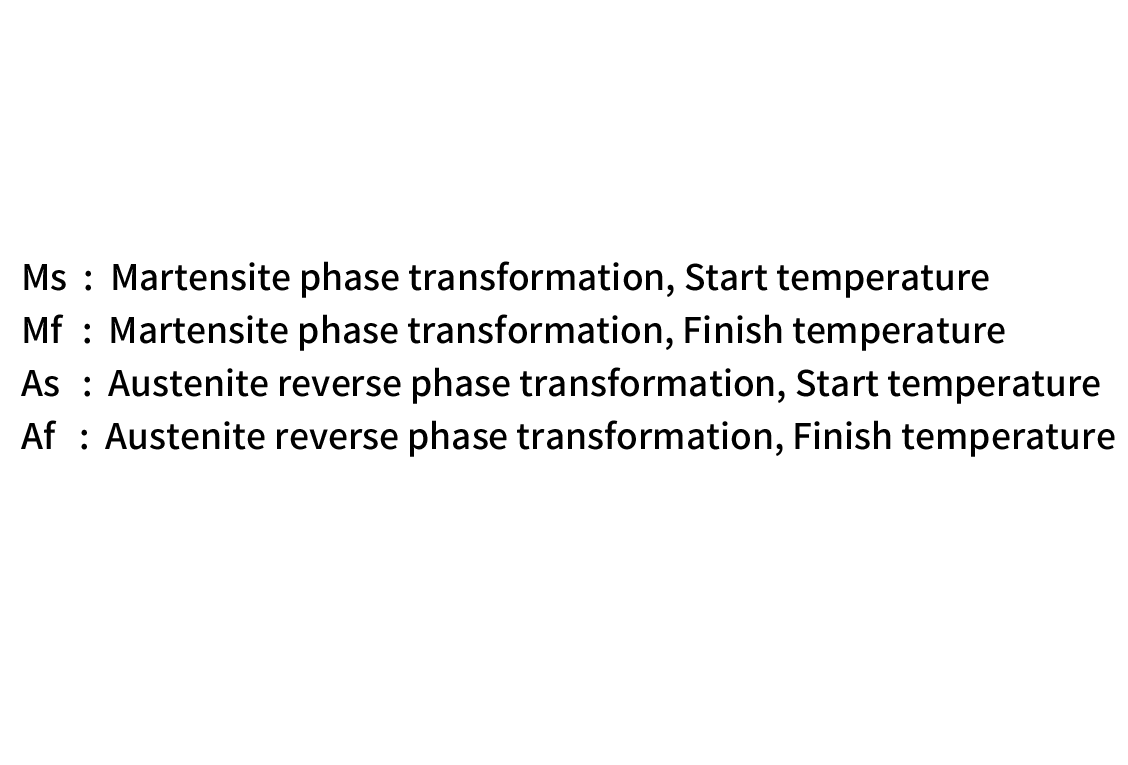

Fig. 5 An example of DSC curve of ingot

Fig. 6 An example of DSC curve of final product