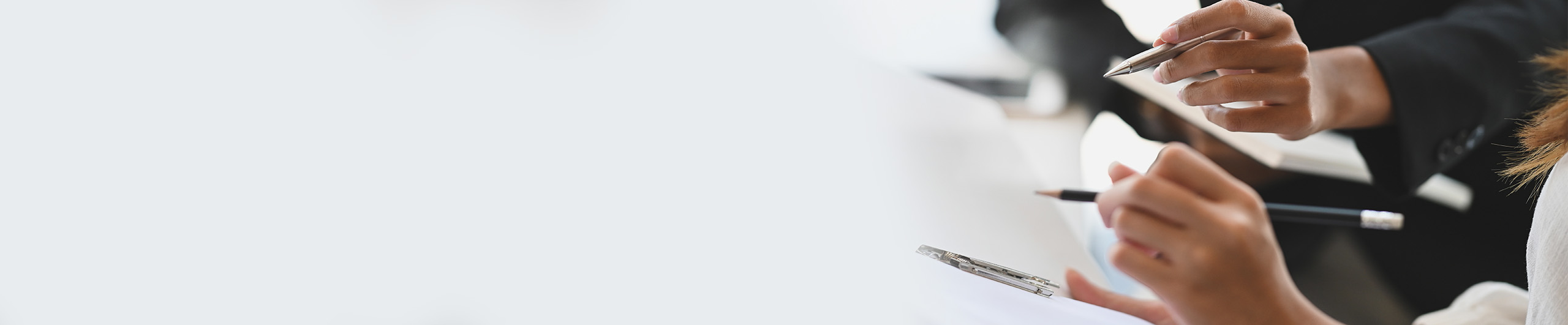
Applications of
Shape Memory Alloy
2. Applications of Shape Memory Alloy
2.1 Applications of Shape memory effect
(1) Shape memory coil spring
A typical application of the shape memory effect is a coil spring as a temperature sensor and actuator. The spring made of Ni-Ti has a sensor function when it reaches or exceeds the shape recovery temperature, or transformation temperature, it generates a driving force as an actuator.
Usually, Ni-Ti springs are used in combination with bias springs(conventional springs) as shown in Fig. 7. When the temperature rises, the Ni-Ti spring generates force and is expanded. When the temperature drops, the force of the bias spring prevails and the unit returns to its original position. This is a two-way operation in response to temperature. In this way, the combination of sensor and actuator eliminates the need for sequencers, wiring, motors, sensors, power supplies, and other parts. Therefore this system can improve reliability, saving space, weight, and reducing costs.
Although shape memory alloys can get two-way effect on the spring as shown in Fig. 7 by special shape memory and deformation processing, they are rarely put to practical use due to their limited characteristics.

Fig. 7 Two-way unit using Ni-Ti spring and bias spring

(a) One-way

(b) Two-way
Fig. 8 Difference of one way and two way shape memory effects
The transverse modulus of elasticity G of Ni-Ti spring changes with temperature. The generated force itself can be calculated in the same way as for ordinary springs, using Hooke’s law and the formula for calculation of the generated force of coil springs, as shown in Fig. 9. From measuring the generated force curve with changing temperature, a temperature vs. transverse modulus curve is created. Using the values, shape memory spring is calculated. Furthermore, a spring unit that combines the Ni-Ti spring with a bias spring can be designed. Shape memory springs have a thermal hysteresis, which is the difference in force generated when the temperature drops and rises. This can be adjusted to some extent by alloy, material, and heat treatment.

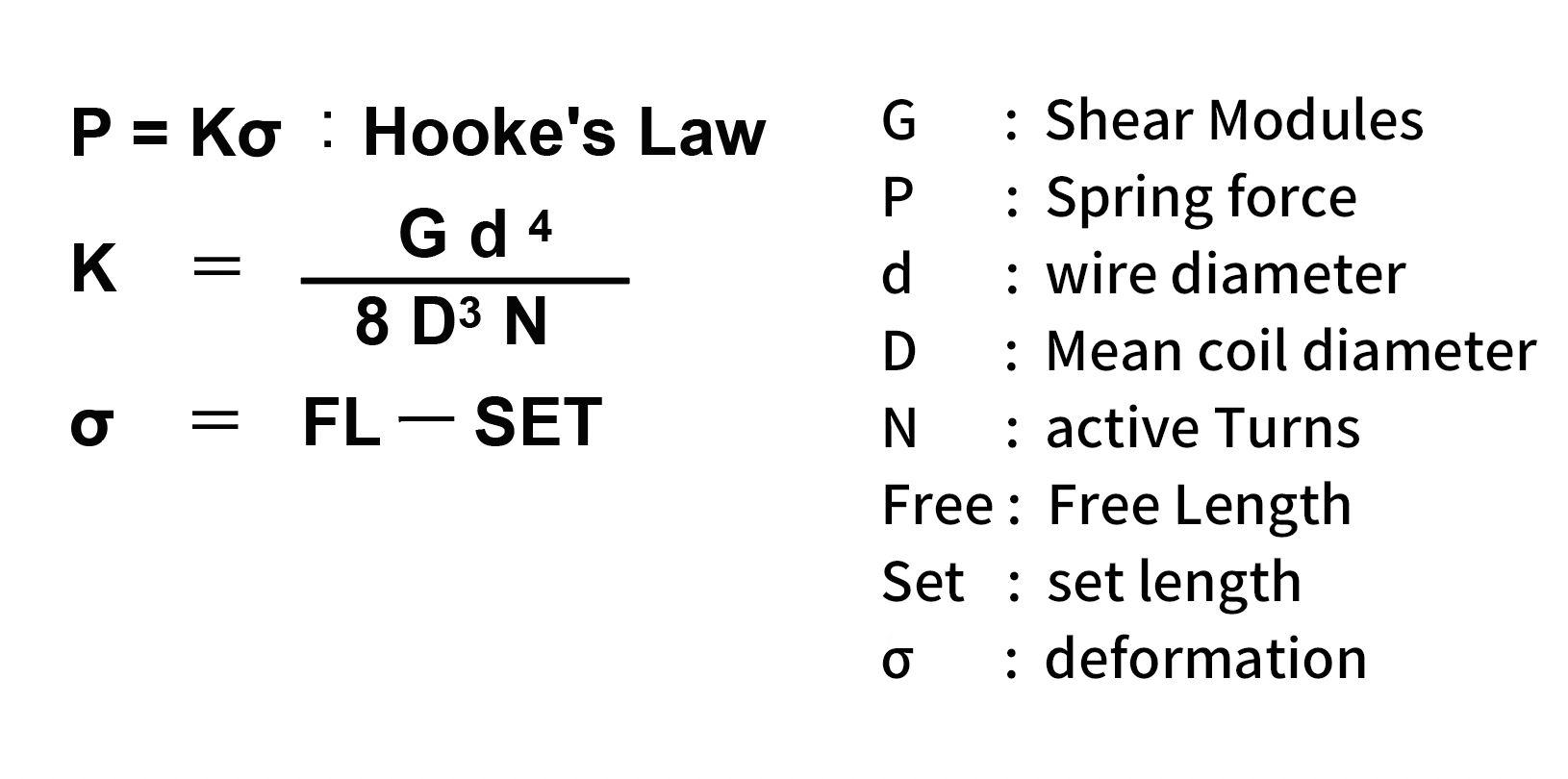
Fig. 9 Calculation of generated force of a coil spring
(2) Ni-Ti electric current actuator
As one of the applications of the shape memory effect, electric current actuators are also being put to practical use. The shape memory effect and the high electrical resistance of Ni-Ti alloy cause the material to self-heat and return to its original shape when energized by current flow. Most of the wires in practical use are relatively thin with a diameter of around 0.1mm. This is because a larger diameter wire has the disadvantage of requiring a larger current and a longer cooling time. It is necessary to optimize overheating, stress, and strain for durability.
2.2 Applications of Super-elasticity
(1) Characteristics of Super-elasticity
Ni-Ti super-elastic alloy shows stress-strain curve as shown in Fig. 10. The characteristics are listed as follows.
- (a) Large strains, as much as 8%, return to the original state.
- (b) The yield stress is relatively high and the strain at that point is large (sometimes over 1%).
- (c) Modulus of elasticity is small (40 to 80 GPa).
- (d) Wide range of constant stress stages under load and unload (4-7%).
- (e) The stress difference between loading and unloading(stress hysteresis) can be adjusted to some extent by alloying and processing heat treatment.
Super-elasticity has been applied to larger number of products than that of the shape memory effect in practical use. The flexible performance of the material, which returns to its original position after bending, has been used in various fields. Recently, it has been applied to medical devices such as guidewires made of Ni-Ti wires and stents made of Ni-Ti tubes by laser cutting. Furthermore, many new medical devices are being developed.
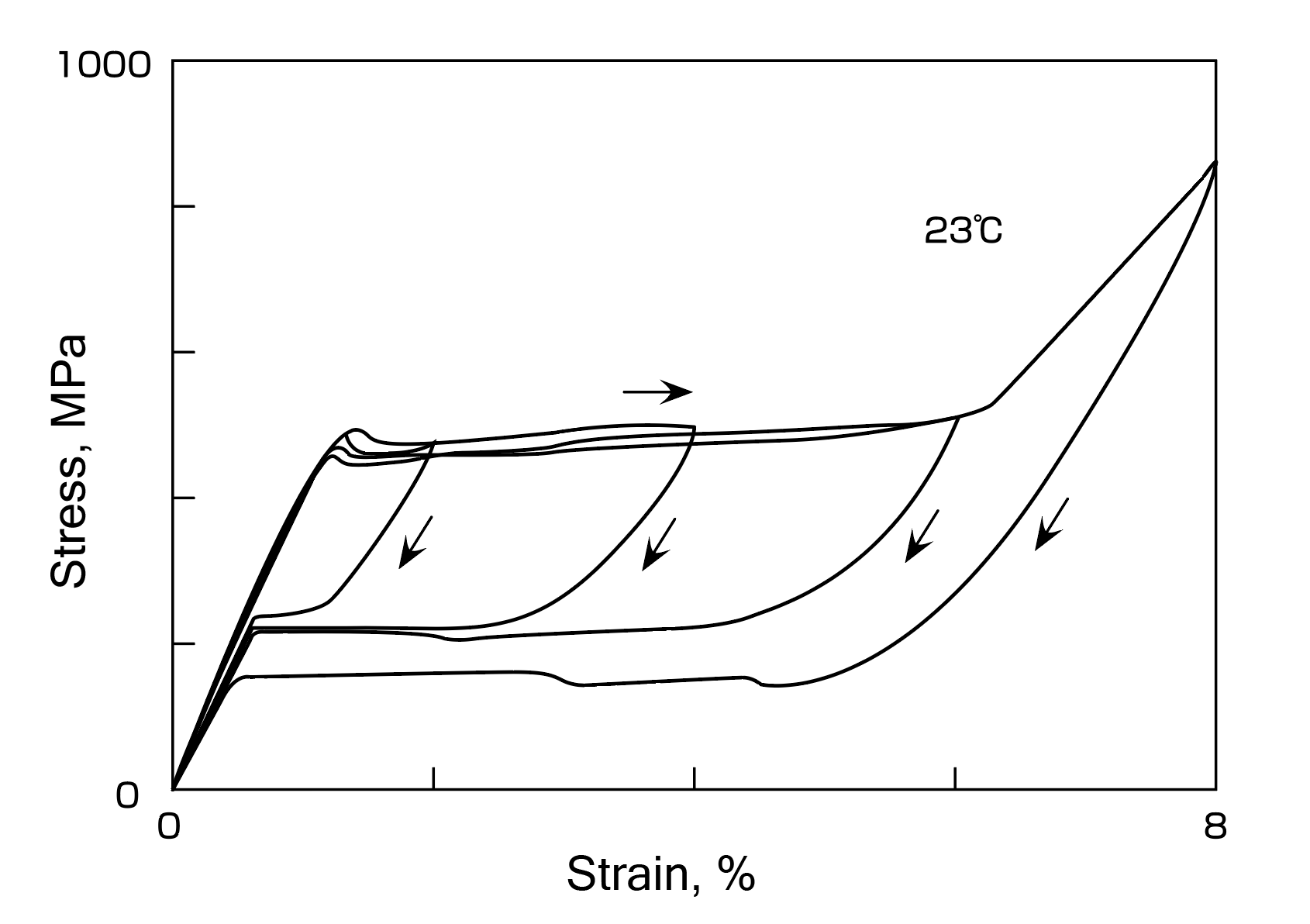
Fig. 10 Typical Stress-Strain curve of Ni-Ti alloy
(2) Mechanical properties of Ni-Ti Super-elasticity
Each characteristic of the stress-strain curve, as defined in ASTM F 2516 for tensile testing of super-elasticity, is shown in Fig. 11.

Fig. 11 Terms Illustrated on typical Stress-Strain curve
of Super-elastic Ni-Ti.
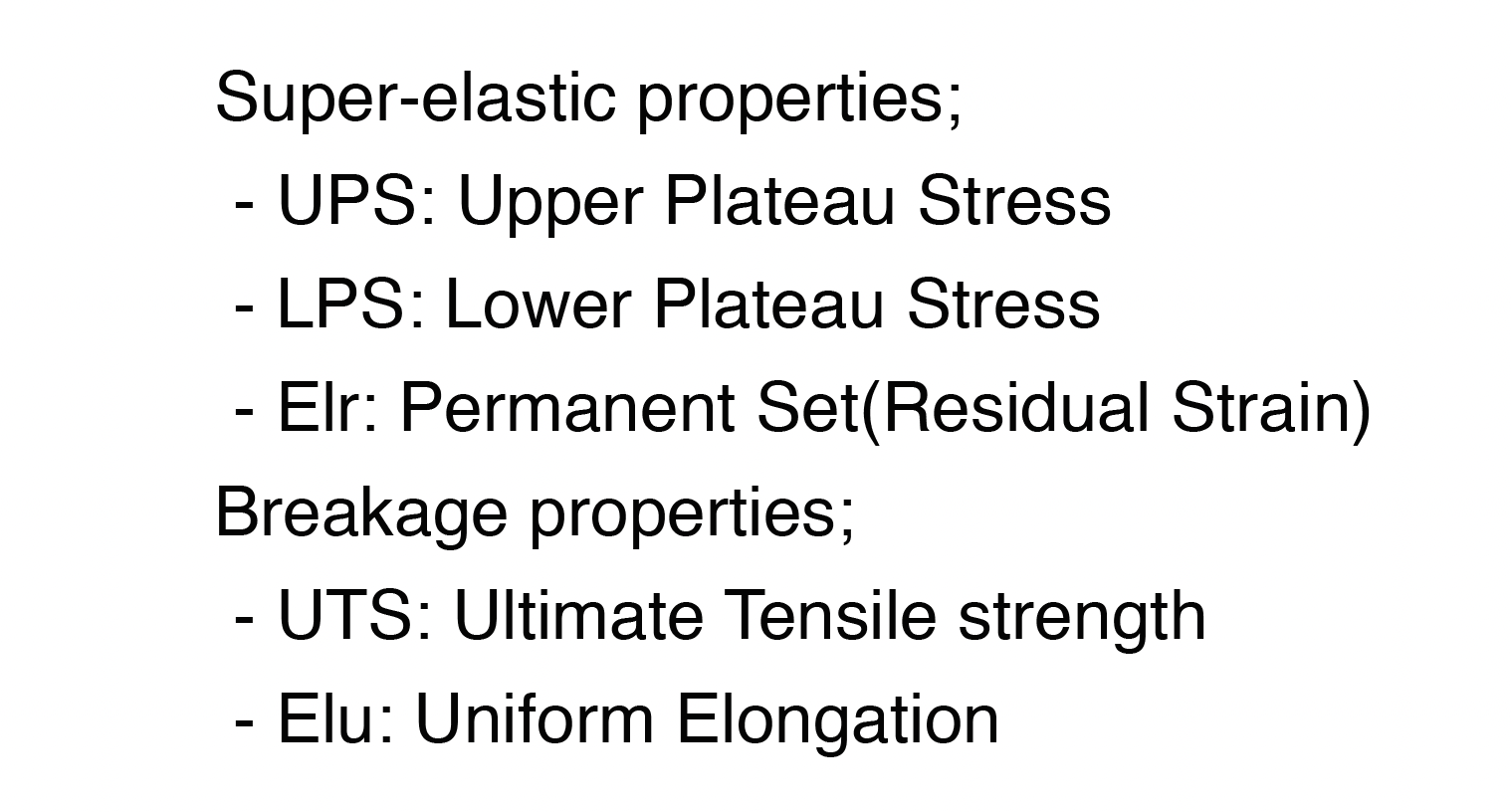
(3) Transformation temperature evaluation method for super-elastic properties in products
The transformation temperature of shape-memory and superelastic alloys can be determined by measuring the heat capacity change due to phase transformation at different temperatures in the ingot or final product using DSC as described above. On the other hand, it is also common to measure the shape recovery with temperature change in the final product, which is defined in ASTM F2082 as the BFR(Bending Free Recovery) testing, as shown in Fig. 12. Since BFR is a mechanical measurement, it is often used because it is close to actual device operation and is easy to measure.

(a) Model of BFR testing equipment

(b) Typical BFR chart of phase transformation
Martensite phase ⇒ Austenite phase
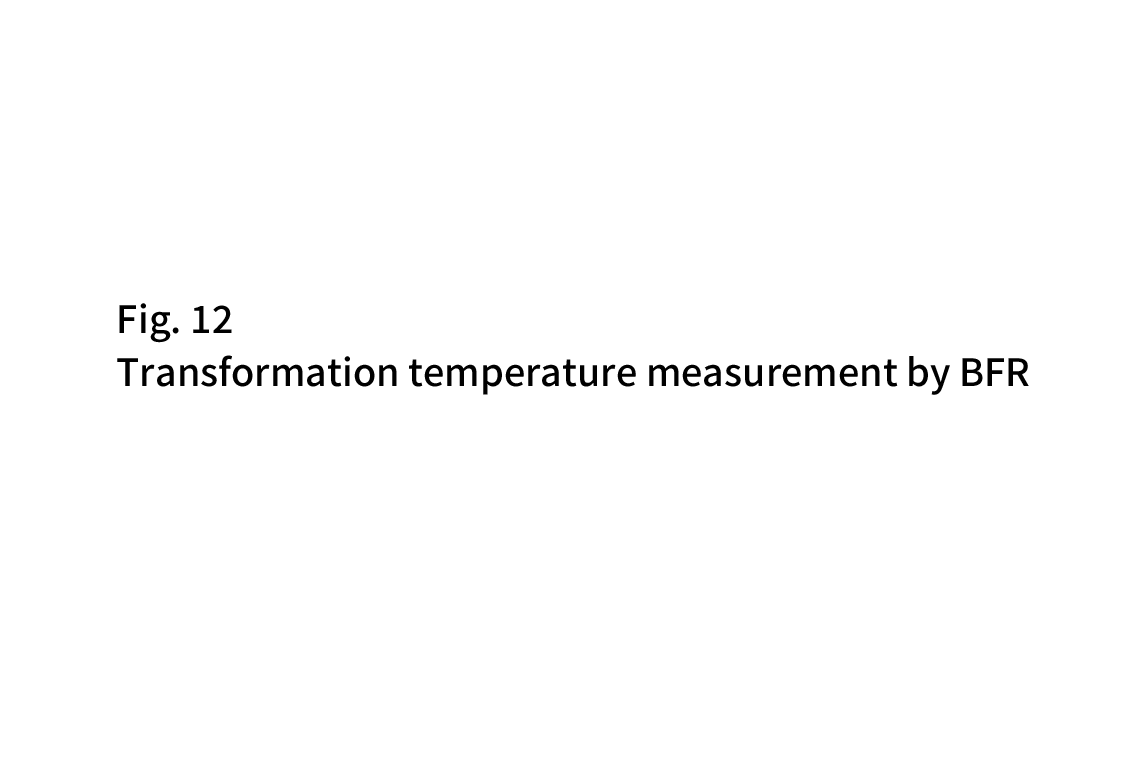

(c) Typical BFR chart of phase transformation
Martensite phase ⇒ R phase ⇒ Austenite phase
(4) Difference between DSC and BFR for Transformation Temperature
As mentioned earlier, DSC measures the calorific value of phase transformation, while BFR measures the amount of mechanical deformation, so their transformation temperatures are not the same, as shown in Fig. 13.
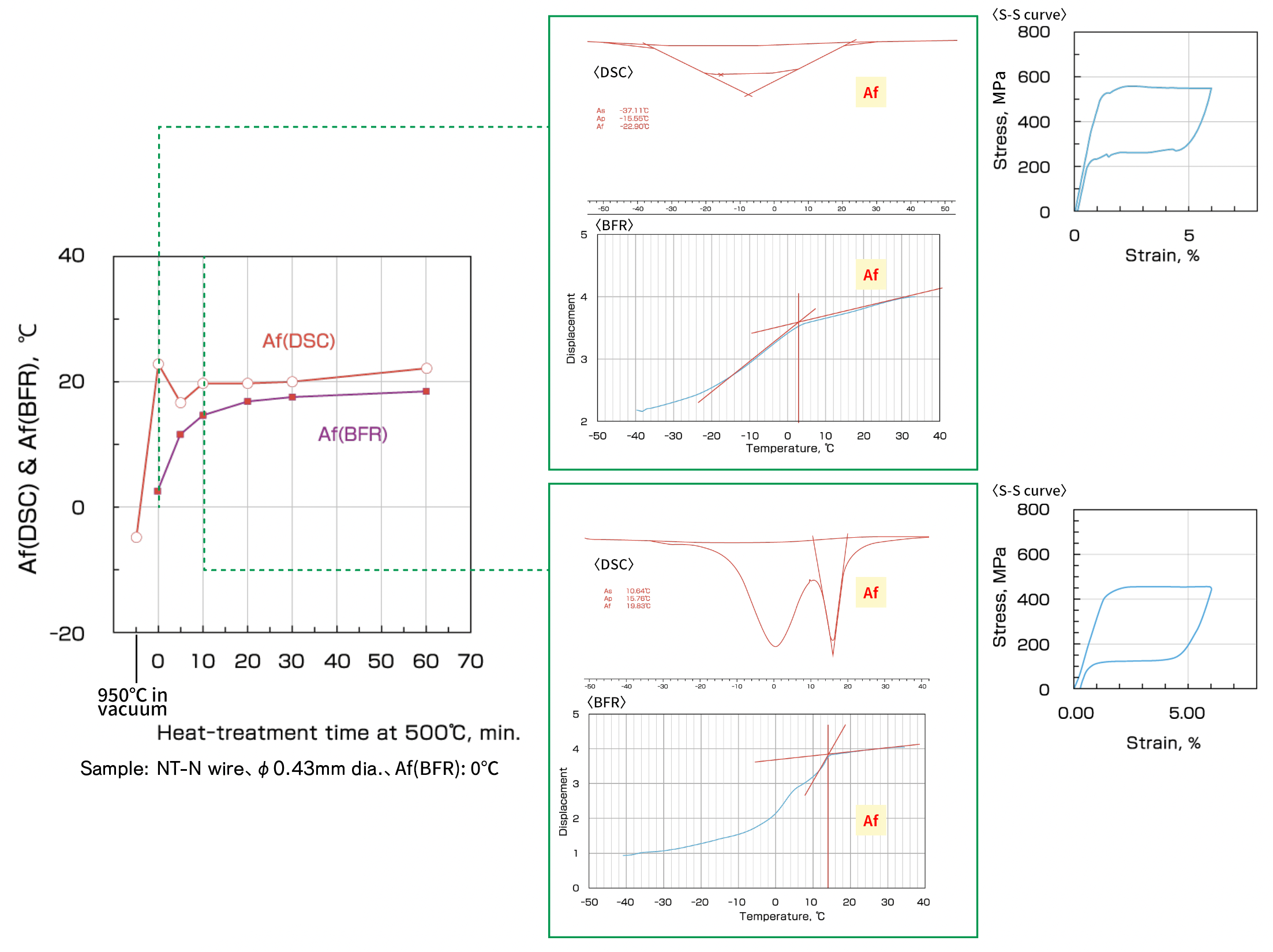
Fig. 13 Comparison of DSC and BFR testings
(5) Effect of Environmental Temperature on Superelastic Properties
The super-elasticity is changed depending on the environmental temperature. Fig. 14 shows the stress-strain curves of Ni-Ti superelastic wire(Alloy type: NT-N, 40% cold worked and heat treated at 500℃) at different environmental temperatures. As shown in Fig. 15, the UPS(Upper Plateau Stress) increases linearly as the environmental temperature increases. This has been proven thermodynamically as the Clausius-Clapeyron relationship. It should be considered when a device is designed.
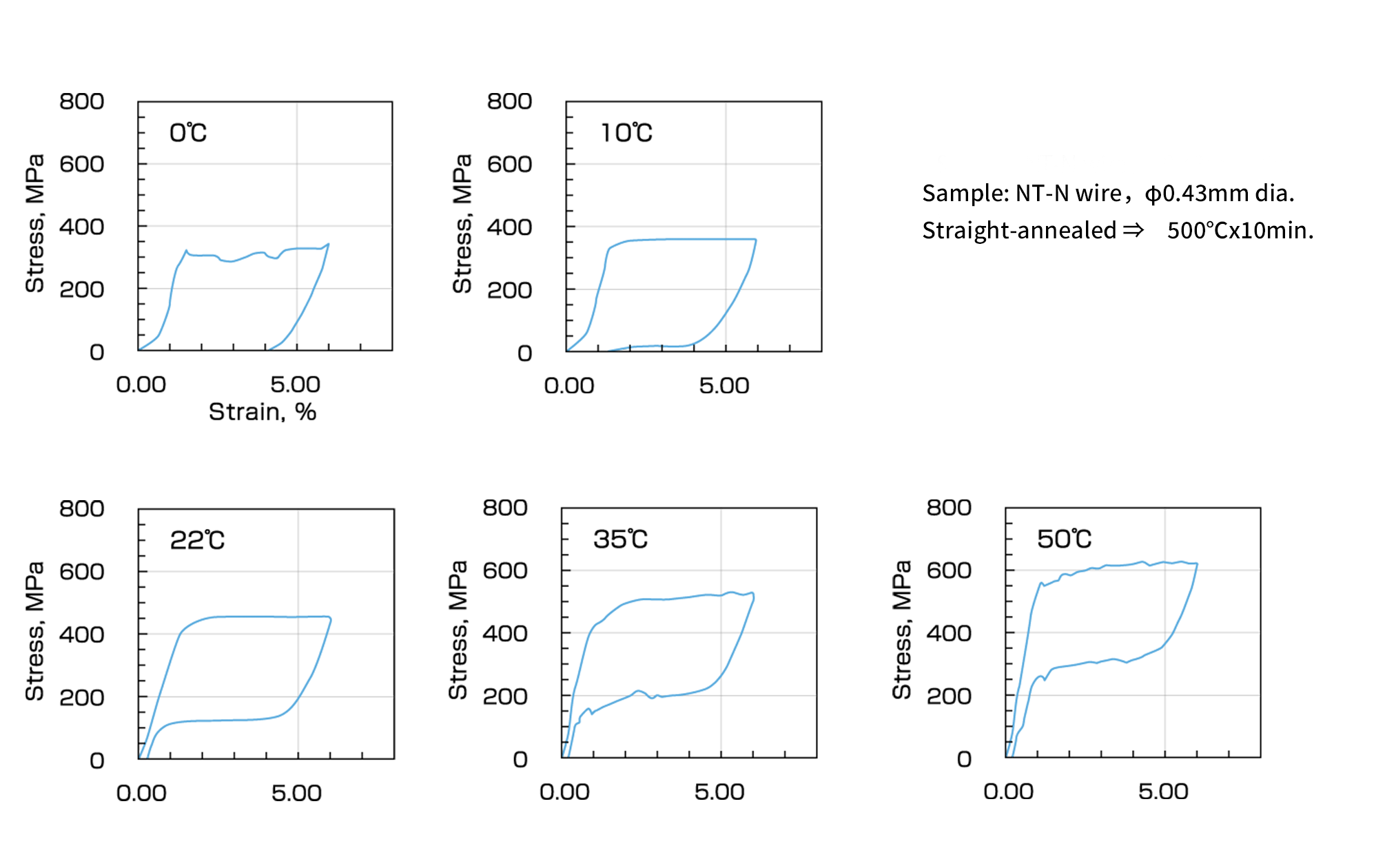
Fig. 14 Change in stress-strain curve with environmental temperature

Fig. 15 Effect of environmental temperature
on Super-elastic mechanical properties
(6) Effect of heat treatment on Super-elastic properties
Fig. 16(a) and (b) show the change in the transformation temperature of NT-N wire after drawing at a cold working rate of 40% and heat treatment. The transformation temperature changes in a complicated manner due to the influence of the R phase transformation described below. The mechanical properties obtained from the stress-strain curves of the same wire are also shown in Fig. 16(c) and (d).

(a) Transformation temperatures (DSC) vs. heat treatment temperature

(b) Transformation temperatures (DSC) vs. heat treatment time

(c) Mechanical properties vs. heat treatment temperature

(d) Mechanical properties vs. heat treatment time
Fig. 16 Influence of heat treatment condition on transformation temperatures and mecanical propeties
Sample: NT-N wire,φ0.43mm dia.,Straight-annealed
(7) Durability evaluation of Super-elasticity
In the application of super-elasticity, in addition to utilizing static properties, repeatable deformation performances are often required. Fig. 17 shows an example of durability data on tensile deformation. In the range where repeated stresses exceed the yield point and stress-induced transformation occurs, the life is at most 10,000 cycles. In the figure, the bending point of the straight line corresponds to the yield stress at which stress-induced transformation occurs. It is believed that the mode of deformation changes over the yield point. In terms of stress, if the yield point of a material is raised and the product is used at a stress below that point, a longer life can be obtained.

Fig. 17 Durability of Super-elastic Ni-Ti wire